Neuron Anatomy And Physiology Exercise 13
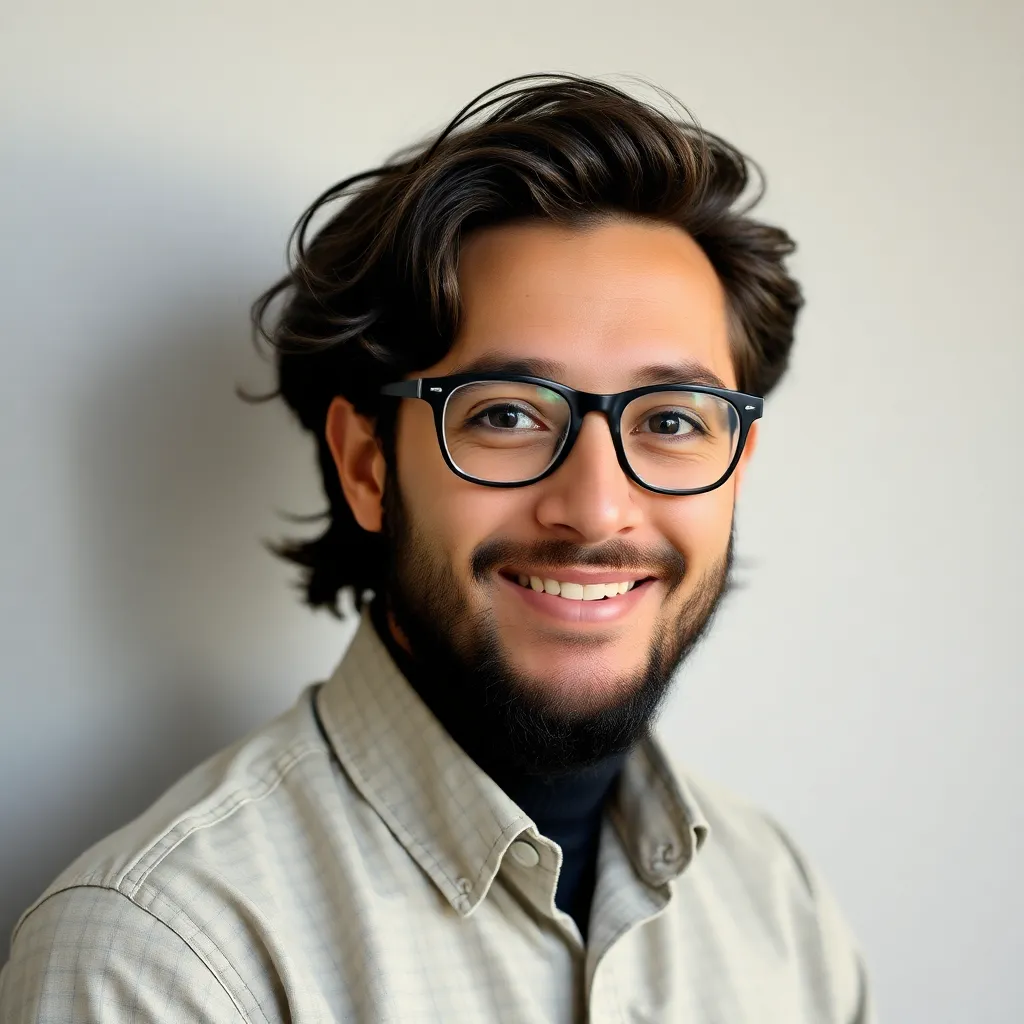
New Snow
Apr 24, 2025 · 8 min read

Table of Contents
Neuron Anatomy and Physiology: Exercise 13 - A Deep Dive into Neural Function
This comprehensive guide delves into the intricacies of neuron anatomy and physiology, expanding upon the concepts introduced in Exercise 13 (assuming a specific curriculum framework). We will explore the structure of a neuron, its functional components, the mechanisms of neural transmission, and the interplay between different neuronal types. This in-depth analysis is designed to solidify your understanding of fundamental neuroscience principles and provide a solid foundation for further study.
I. Neuron Anatomy: The Building Blocks of the Nervous System
Neurons, the fundamental units of the nervous system, are highly specialized cells responsible for receiving, processing, and transmitting information. Their complex structure is intimately linked to their function.
A. The Soma (Cell Body): The Neuron's Control Center
The soma, or cell body, contains the neuron's nucleus and essential organelles, including the ribosomes, endoplasmic reticulum, and Golgi apparatus. These structures are responsible for protein synthesis, crucial for the neuron's maintenance and function. The soma also integrates signals received from dendrites, ultimately determining whether to fire an action potential. The health and integrity of the soma are vital for the neuron's overall viability.
B. Dendrites: The Receiving Antennas
Dendrites are branched extensions of the soma that act as the primary recipients of signals from other neurons. Their extensive branching pattern maximizes the surface area available for synaptic input. Specialized receptors on the dendritic membrane bind neurotransmitters, initiating a cascade of events that either excite or inhibit the neuron. The complexity of dendritic branching varies considerably depending on the neuron's type and location in the nervous system.
C. Axon: The Information Highway
The axon is a long, slender projection extending from the soma, responsible for transmitting signals over long distances. The axon's length can vary dramatically, from a few micrometers to over a meter in some cases. The axon is covered by a myelin sheath in many neurons, which acts as an insulator and significantly increases the speed of signal transmission. The gaps between myelin segments are known as Nodes of Ranvier, where action potentials are regenerated.
D. Axon Terminals (Synaptic Boutons): The Communication Hubs
At its distal end, the axon branches into numerous axon terminals, also known as synaptic boutons. These terminals form synapses with other neurons or target cells, allowing for communication via neurotransmitters. The synapse is the crucial junction where information is passed from one neuron to another. The release of neurotransmitters from synaptic vesicles into the synaptic cleft is a highly regulated process crucial for neural signaling.
II. Neuron Physiology: The Mechanisms of Neural Communication
The physiology of a neuron encompasses the complex processes involved in receiving, integrating, and transmitting information. Understanding these mechanisms is essential for comprehending the workings of the nervous system as a whole.
A. The Resting Membrane Potential: A State of Readiness
In its resting state, the neuron maintains a negative membrane potential, typically around -70 mV. This potential difference is due to the unequal distribution of ions across the neuronal membrane, maintained by ion pumps and channels. The sodium-potassium pump plays a crucial role, actively transporting sodium ions out of the cell and potassium ions into the cell, contributing to the negative resting potential.
B. Graded Potentials: Summing the Input
Dendrites receive signals from other neurons in the form of graded potentials. These potentials are changes in membrane potential that vary in amplitude depending on the strength of the stimulus. Excitatory postsynaptic potentials (EPSPs) depolarize the membrane, bringing it closer to the threshold for firing an action potential, while inhibitory postsynaptic potentials (IPSPs) hyperpolarize the membrane, moving it further from the threshold. The soma integrates these EPSPs and IPSPs; if the sum reaches the threshold potential, an action potential is triggered.
C. The Action Potential: The All-or-None Signal
The action potential is a rapid, transient reversal of the membrane potential, propagating along the axon without decrement. It's an all-or-none event, meaning it either occurs fully or not at all. The rising phase of the action potential is driven by the influx of sodium ions through voltage-gated sodium channels, while the falling phase is due to the efflux of potassium ions through voltage-gated potassium channels. The refractory period following an action potential ensures unidirectional propagation of the signal along the axon.
D. Myelin and Saltatory Conduction: Speeding Up Transmission
Myelin, a fatty insulating layer surrounding many axons, significantly increases the speed of action potential propagation. Instead of propagating continuously along the axon, the action potential "jumps" from one Node of Ranvier to the next, a process called saltatory conduction. This mechanism dramatically accelerates signal transmission compared to unmyelinated axons.
E. Neurotransmission at the Synapse: Chemical Communication
At the synapse, the arrival of an action potential at the axon terminal triggers the release of neurotransmitters. These chemicals diffuse across the synaptic cleft and bind to receptors on the postsynaptic membrane, initiating a postsynaptic potential (either EPSP or IPSP). Neurotransmitter release is a precisely regulated process, involving the fusion of synaptic vesicles with the presynaptic membrane and the subsequent diffusion of neurotransmitters.
III. Types of Neurons and Their Functional Roles
The nervous system contains a diverse array of neurons, each specialized for a particular function. Understanding these different types is crucial for comprehending the complexity of neural circuits.
A. Sensory Neurons (Afferent Neurons): Transmitting Information from the Periphery
Sensory neurons are responsible for transmitting information from sensory receptors to the central nervous system (CNS). They detect stimuli such as touch, temperature, pain, and light, converting them into electrical signals that are relayed to the brain and spinal cord. These neurons have specialized receptors on their peripheral endings that are sensitive to specific types of stimuli.
B. Motor Neurons (Efferent Neurons): Commanding Muscle Action
Motor neurons transmit signals from the CNS to muscles and glands, causing them to contract or secrete substances. These neurons are responsible for voluntary and involuntary movements, as well as the regulation of various physiological processes. Their axons extend from the CNS to the periphery, forming neuromuscular junctions with muscle fibers.
C. Interneurons: Integrating Information Within the CNS
Interneurons are located entirely within the CNS and act as intermediaries between sensory and motor neurons. They process and integrate information from various sources, coordinating complex neural circuits. The vast majority of neurons in the CNS are interneurons, playing a critical role in information processing, decision-making, and learning.
IV. Exercise 13: Applying the Knowledge (Hypothetical Examples)
Let's assume Exercise 13 involves solving problems related to neuron anatomy and physiology. Here are some hypothetical examples of questions and their explanations based on the concepts discussed above:
Example 1: A drug blocks voltage-gated sodium channels. What effect would this have on action potential generation and propagation?
Explanation: Blocking voltage-gated sodium channels would prevent the influx of sodium ions during the rising phase of the action potential. Consequently, the neuron would be unable to depolarize sufficiently to reach the threshold, and action potentials would not be generated. This would effectively shut down neural signaling.
Example 2: Describe the events that occur at a synapse following the arrival of an action potential at the axon terminal.
Explanation: The arrival of an action potential at the axon terminal triggers the opening of voltage-gated calcium channels. Calcium influx causes synaptic vesicles containing neurotransmitters to fuse with the presynaptic membrane and release their contents into the synaptic cleft. Neurotransmitters then diffuse across the cleft and bind to receptors on the postsynaptic membrane, inducing a postsynaptic potential (EPSP or IPSP).
Example 3: Compare and contrast the structures and functions of sensory neurons and motor neurons.
Explanation: Sensory neurons transmit information from sensory receptors to the CNS, while motor neurons transmit signals from the CNS to muscles and glands. Sensory neurons typically have long dendrites and short axons, whereas motor neurons have long axons and shorter dendrites. Sensory neurons have specialized receptors on their peripheral endings, while motor neurons form neuromuscular junctions with muscle fibers.
Example 4: Explain the role of myelin in increasing the speed of action potential propagation.
Explanation: Myelin acts as an insulator, preventing ion leakage across the axonal membrane. This allows the action potential to propagate much faster through saltatory conduction – “jumping” from one Node of Ranvier to the next, rather than traveling continuously along the axon.
Example 5: How would damage to the soma of a neuron affect its function?
Explanation: The soma houses the neuron's nucleus and essential organelles. Damage to the soma would disrupt protein synthesis, compromise metabolic processes, and ultimately lead to neuronal dysfunction or death. This would disrupt signal integration and transmission.
V. Further Exploration: Delving Deeper into Neuroscience
This detailed exploration of neuron anatomy and physiology provides a robust foundation for further study. Exploring specific neurotransmitters, neural circuits, and the complex interactions within the nervous system are all crucial steps in expanding your understanding of neuroscience. Consider investigating topics like:
- Specific Neurotransmitters: The roles of different neurotransmitters (e.g., glutamate, GABA, dopamine, serotonin) in various neural pathways and their implications for behavior and disease.
- Neural Circuits: How neurons are organized into networks to perform specific functions, such as processing sensory information or generating motor commands.
- Neurodegenerative Diseases: How dysfunction or damage to neurons contribute to neurological disorders like Alzheimer's disease, Parkinson's disease, and multiple sclerosis.
- Neuroplasticity: The brain's ability to reorganize itself throughout life, adapting to changes and forming new connections.
By continuously exploring these advanced concepts and applying the fundamental principles discussed here, you can build a comprehensive understanding of the remarkable complexity and elegance of the nervous system. This deep dive into Exercise 13's concepts forms a solid base for more sophisticated neuroscience investigations. Remember to actively engage with the material, practicing problem-solving and critical thinking to solidify your knowledge and prepare for future studies.
Latest Posts
Latest Posts
-
Which Data Type Can Only Be Classified As Text
Apr 25, 2025
-
A Diagnosis Of Type 1 Diabetes Mellitus Implies That
Apr 25, 2025
-
Think And Grow Rich Pdf Napoleon Hill
Apr 25, 2025
-
Sunday In The Park By Bel Kaufman
Apr 25, 2025
-
According To Zemkes Four Generations Of Workers The Millennials
Apr 25, 2025
Related Post
Thank you for visiting our website which covers about Neuron Anatomy And Physiology Exercise 13 . We hope the information provided has been useful to you. Feel free to contact us if you have any questions or need further assistance. See you next time and don't miss to bookmark.