Experiment 13 The Geometrical Structure Of Molecules Answers
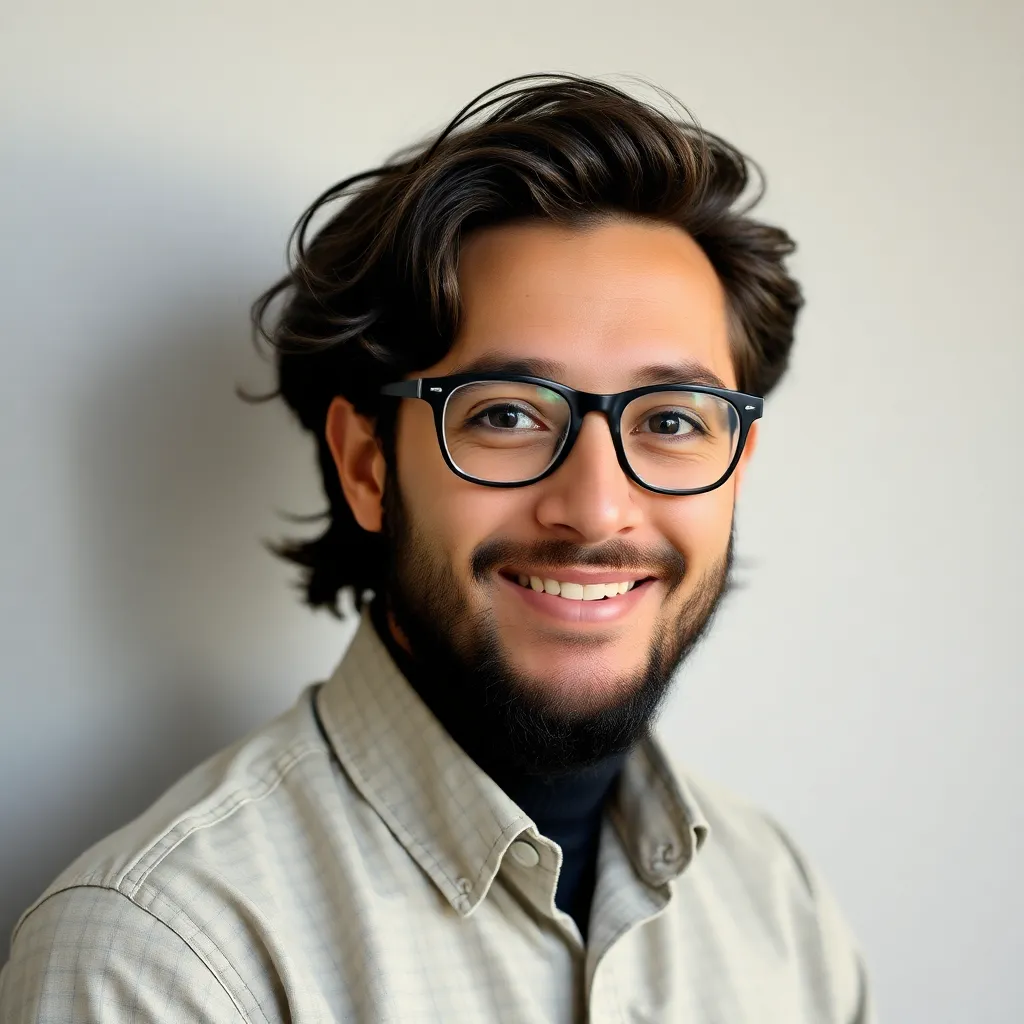
New Snow
Apr 23, 2025 · 5 min read

Table of Contents
Experiment 13: The Geometrical Structure of Molecules – A Deep Dive into Answers
Understanding molecular geometry is crucial in chemistry, impacting reactivity, polarity, and physical properties. Experiment 13, typically found in introductory chemistry courses, aims to solidify this understanding through hands-on exploration. This comprehensive guide delves into the concepts behind Experiment 13, providing detailed answers and explanations to common questions and challenges students encounter. We'll explore the VSEPR theory, analyze different molecular shapes, and examine the impact of lone pairs and multiple bonds.
Understanding VSEPR Theory: The Foundation of Molecular Geometry
The Valence Shell Electron Pair Repulsion (VSEPR) theory forms the cornerstone of predicting molecular geometries. This theory postulates that electron pairs, both bonding and non-bonding (lone pairs), repel each other and arrange themselves to minimize this repulsion. This arrangement dictates the overall shape of the molecule. The key to understanding Experiment 13 lies in mastering VSEPR.
Steric Number: The Key to Predicting Geometry
The steric number is the sum of the number of atoms bonded to the central atom and the number of lone pairs on the central atom. This number is critical in determining the electron-pair geometry. Different steric numbers lead to different electron-pair geometries, as detailed below:
- Steric Number 2: Linear electron-pair geometry (e.g., BeCl₂).
- Steric Number 3: Trigonal planar electron-pair geometry (e.g., BF₃).
- Steric Number 4: Tetrahedral electron-pair geometry (e.g., CH₄).
- Steric Number 5: Trigonal bipyramidal electron-pair geometry (e.g., PCl₅).
- Steric Number 6: Octahedral electron-pair geometry (e.g., SF₆).
It's crucial to note that the molecular geometry, which describes the arrangement of atoms only, might differ from the electron-pair geometry if lone pairs are present. Lone pairs occupy more space than bonding pairs, influencing the overall molecular shape.
Common Molecular Geometries and Their Characteristics
Experiment 13 likely covers several common molecular geometries. Let's examine them in detail:
1. Linear Geometry (Steric Number 2)
Molecules with a steric number of 2 exhibit a linear geometry. The bond angle is 180°. Examples include BeCl₂ and CO₂. The presence of lone pairs on the central atom is absent in linear molecules. In Experiment 13, understanding the absence of lone pair influence is crucial for accurately predicting the geometry.
2. Trigonal Planar Geometry (Steric Number 3)
With a steric number of 3 and no lone pairs, molecules adopt a trigonal planar geometry. The bond angles are approximately 120°. BF₃ is a classic example. The introduction of a lone pair would distort this geometry, leading to a bent shape (as seen below).
3. Tetrahedral Geometry (Steric Number 4)
The tetrahedral geometry, characterized by a steric number of 4 and no lone pairs, is extremely common. Methane (CH₄) is the quintessential example. Bond angles are approximately 109.5°. The presence of one or more lone pairs alters this geometry significantly, leading to variations like trigonal pyramidal and bent shapes.
4. Trigonal Pyramidal Geometry (Steric Number 4 with One Lone Pair)
When a central atom with a steric number of 4 has one lone pair, the molecular geometry becomes trigonal pyramidal. Ammonia (NH₃) is the prime example. The lone pair pushes the bonding pairs closer together, resulting in bond angles slightly less than 109.5° (typically around 107°). This deviation is a key observation in Experiment 13.
5. Bent Geometry (Steric Number 4 with Two Lone Pairs)
With two lone pairs on a central atom having a steric number of 4, the molecular geometry is bent. Water (H₂O) perfectly illustrates this. The two lone pairs significantly repel the bonding pairs, reducing the bond angle to approximately 104.5°. This considerable reduction from the ideal tetrahedral angle highlights the strong influence of lone pairs.
6. Trigonal Bipyramidal Geometry (Steric Number 5)
Molecules with a steric number of 5 and no lone pairs exhibit a trigonal bipyramidal geometry. Phosphorus pentachloride (PCl₅) is a good example. This geometry involves three equatorial positions and two axial positions, with different bond angles. The addition of lone pairs significantly alters this structure.
7. Octahedral Geometry (Steric Number 6)
With a steric number of 6 and no lone pairs, molecules adopt an octahedral geometry. Sulfur hexafluoride (SF₆) is a typical example. This symmetrical geometry has bond angles of 90° and 180°. Lone pairs significantly distort this geometry.
The Impact of Multiple Bonds
Experiment 13 should also address the influence of multiple bonds (double and triple bonds) on molecular geometry. Multiple bonds occupy more space than single bonds, thus influencing the arrangement of other atoms and lone pairs. They exert a stronger repulsive force, potentially affecting bond angles.
Analyzing Experimental Data in Experiment 13
Experiment 13 likely involves building molecular models, using software simulations, or analyzing experimental data to predict and confirm molecular geometries. Analyzing this data correctly is critical. Here are some key considerations:
- Accurate Lewis Structures: Begin by drawing accurate Lewis structures for each molecule. This step determines the steric number and the number of lone pairs.
- Electron-Pair Geometry vs. Molecular Geometry: Remember the distinction between these two concepts. Lone pairs affect the molecular geometry but not the electron-pair geometry.
- Bond Angles: Pay close attention to the bond angles. Deviations from ideal angles strongly suggest the presence and influence of lone pairs.
- Polarity: Consider the polarity of individual bonds and the overall molecular polarity. Molecular geometry significantly impacts the overall polarity.
Advanced Concepts and Applications
Experiment 13 might touch upon more advanced concepts such as:
- Hybridization: Understanding orbital hybridization (sp, sp², sp³, etc.) provides a more in-depth view of bonding and geometry.
- Bond Length and Strength: Molecular geometry impacts bond lengths and the strengths of bonds.
- Isomerism: Geometric isomerism (cis-trans isomerism) arises from the different spatial arrangements of atoms around a double bond.
Conclusion: Mastering Molecular Geometry
Experiment 13 serves as a crucial stepping stone in understanding molecular geometry. By mastering the concepts of VSEPR theory, recognizing the influence of lone pairs and multiple bonds, and carefully analyzing experimental data, students can build a solid foundation in this critical area of chemistry. This comprehensive guide provides detailed answers and explanations to help navigate the complexities of Experiment 13 and its associated concepts, fostering a deeper understanding of the fundamental principles governing the three-dimensional structure of molecules. Remember, practice and careful observation are key to mastering the art of predicting molecular geometry. By systematically working through different examples and analyzing the impact of lone pairs and multiple bonds, you can solidify your understanding and excel in your studies.
Latest Posts
Latest Posts
-
Titration Of Fruit Juice Lab Answers
Apr 23, 2025
-
1455 Market Street San Francisco Cash App
Apr 23, 2025
-
How Has Diversity Influenced The Humanities
Apr 23, 2025
-
Consumption Tax Sales In States Answer Key
Apr 23, 2025
-
Creating Chains And Webs To Model Ecological Relationships Answers
Apr 23, 2025
Related Post
Thank you for visiting our website which covers about Experiment 13 The Geometrical Structure Of Molecules Answers . We hope the information provided has been useful to you. Feel free to contact us if you have any questions or need further assistance. See you next time and don't miss to bookmark.