Dna Biology And Technology Transcription Translation And Mutation
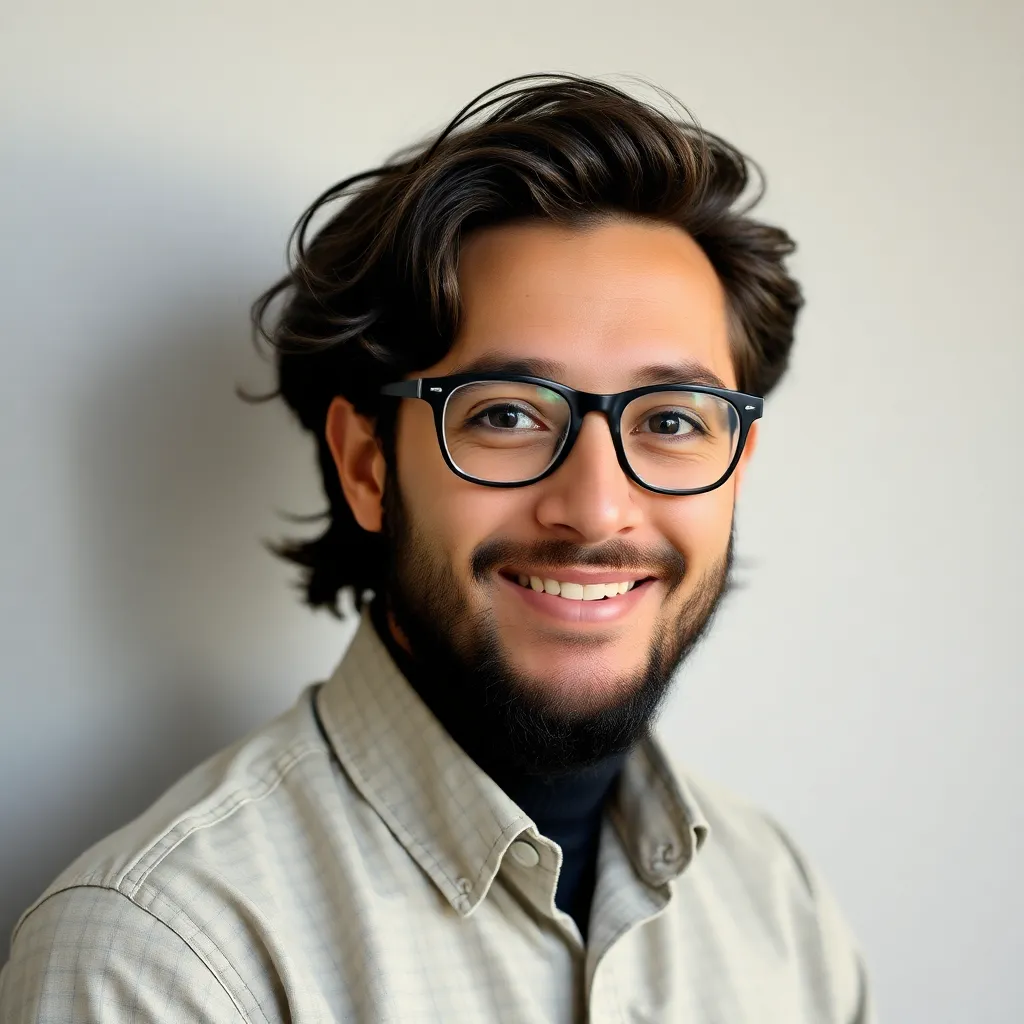
New Snow
Apr 24, 2025 · 7 min read

Table of Contents
DNA Biology and Technology: Transcription, Translation, and Mutation
DNA, the blueprint of life, holds the instructions for building and maintaining all living organisms. Understanding DNA, its processes, and the technologies surrounding it is crucial for advancements in medicine, agriculture, and many other fields. This article delves deep into the fascinating world of DNA biology, focusing on transcription, translation, and mutation, and exploring the technological applications arising from this knowledge.
The Central Dogma of Molecular Biology: From DNA to Protein
The central dogma describes the flow of genetic information within a biological system. It posits that DNA is transcribed into RNA, which is then translated into proteins. These proteins perform a vast array of functions within the cell, ultimately determining an organism's traits and characteristics. Let's explore each stage in detail:
DNA: The Master Blueprint
Deoxyribonucleic acid (DNA) is a double-stranded helix composed of nucleotides. Each nucleotide consists of a deoxyribose sugar, a phosphate group, and one of four nitrogenous bases: adenine (A), guanine (G), cytosine (C), and thymine (T). The bases pair specifically – A with T, and G with C – through hydrogen bonds, forming the characteristic double helix structure. The sequence of these bases along the DNA molecule encodes the genetic information. This information is organized into genes, which are specific segments of DNA that code for functional RNA molecules, typically messenger RNA (mRNA) which then codes for proteins.
Transcription: From DNA to RNA
Transcription is the process of creating an RNA molecule from a DNA template. It occurs within the nucleus of eukaryotic cells and the cytoplasm of prokaryotic cells. The enzyme responsible for transcription is RNA polymerase. This enzyme binds to a specific region of the DNA called the promoter, unwinds the DNA double helix, and begins synthesizing a complementary RNA molecule. Instead of thymine (T), RNA uses uracil (U), which pairs with adenine (A). The resulting RNA molecule, primarily mRNA, carries the genetic code from the DNA to the ribosome, the site of protein synthesis.
Key players in transcription:
- RNA Polymerase: The enzyme that synthesizes the RNA molecule.
- Promoter: A specific DNA sequence that signals the start of transcription.
- Terminator: A DNA sequence that signals the end of transcription.
- Transcription factors: Proteins that regulate the rate of transcription.
RNA Processing (in Eukaryotes): Preparing the mRNA
In eukaryotic cells, the newly synthesized pre-mRNA undergoes several processing steps before it can be translated into protein:
- Capping: A modified guanine nucleotide is added to the 5' end of the pre-mRNA, protecting it from degradation and aiding in ribosome binding.
- Splicing: Non-coding regions of the pre-mRNA, called introns, are removed, and the coding regions, called exons, are joined together. This process is crucial for generating mature mRNA with the correct coding sequence.
- Polyadenylation: A poly(A) tail, a long string of adenine nucleotides, is added to the 3' end of the mRNA, further protecting it from degradation and aiding in its transport out of the nucleus.
Translation: From RNA to Protein
Translation is the process of synthesizing a polypeptide chain (protein) from an mRNA template. It takes place at the ribosome, a complex molecular machine composed of ribosomal RNA (rRNA) and proteins. The mRNA molecule binds to the ribosome, and the ribosome moves along the mRNA, reading the genetic code in three-nucleotide units called codons. Each codon specifies a particular amino acid. Transfer RNA (tRNA) molecules, each carrying a specific amino acid, recognize and bind to their corresponding codons on the mRNA. The ribosome facilitates the formation of peptide bonds between the amino acids, creating a growing polypeptide chain. Once the ribosome reaches a stop codon, the polypeptide chain is released, and it folds into a functional protein.
Key players in translation:
- Ribosomes: The site of protein synthesis.
- mRNA: Carries the genetic code from DNA.
- tRNA: Carries amino acids to the ribosome.
- Aminoacyl-tRNA synthetases: Enzymes that attach amino acids to their corresponding tRNA molecules.
- Codons: Three-nucleotide sequences on mRNA that specify amino acids.
- Anticodons: Three-nucleotide sequences on tRNA that are complementary to codons.
DNA Technology: Harnessing the Power of Genes
Our understanding of DNA has led to the development of powerful technologies that allow us to manipulate and analyze DNA. These technologies have revolutionized various fields, including medicine, agriculture, and forensics.
Polymerase Chain Reaction (PCR): Amplifying DNA
PCR is a revolutionary technique that allows scientists to amplify specific DNA sequences exponentially. Using a heat-stable DNA polymerase, PCR generates millions of copies of a target DNA sequence from a small starting sample. This is crucial for various applications, including disease diagnosis, genetic testing, and forensic investigations.
DNA Sequencing: Reading the Genetic Code
DNA sequencing technologies enable us to determine the precise order of nucleotides in a DNA molecule. Various methods exist, ranging from Sanger sequencing to next-generation sequencing (NGS) techniques, which allow for the rapid and cost-effective sequencing of entire genomes. This information is crucial for understanding genetic variation, identifying disease-causing mutations, and studying evolutionary relationships.
CRISPR-Cas9 Gene Editing: Precise Genome Modification
CRISPR-Cas9 is a powerful gene-editing technology that allows scientists to precisely modify DNA sequences. This technology utilizes a guide RNA molecule to target a specific DNA sequence and the Cas9 enzyme to cut the DNA at that location. The cell's natural repair mechanisms can then be used to introduce changes into the DNA, potentially correcting genetic defects or introducing new traits. This technology holds immense promise for treating genetic diseases and developing new therapies.
Gene Cloning and Expression: Producing Proteins of Interest
Gene cloning involves isolating a specific gene and inserting it into a vector, such as a plasmid, which can then be introduced into a host organism. This allows for the large-scale production of the protein encoded by the gene. This technique has been used to produce various important proteins, including insulin, human growth hormone, and many therapeutic antibodies.
Mutations: Changes in the Genetic Code
Mutations are changes in the DNA sequence that can have various effects on the organism. They can be caused by errors during DNA replication or by exposure to mutagens, such as radiation or certain chemicals.
Types of Mutations:
- Point mutations: These involve changes in a single nucleotide base. They can be:
- Silent mutations: Do not alter the amino acid sequence due to redundancy in the genetic code.
- Missense mutations: Change a single amino acid in the protein sequence, potentially altering its function.
- Nonsense mutations: Introduce a premature stop codon, resulting in a truncated and often non-functional protein.
- Frameshift mutations: These involve the insertion or deletion of nucleotides that are not multiples of three. They shift the reading frame of the codons, altering the amino acid sequence downstream of the mutation, often leading to non-functional proteins.
- Chromosomal mutations: These involve larger-scale changes in the chromosome structure, such as deletions, duplications, inversions, and translocations. These can have severe consequences, often leading to developmental abnormalities or genetic diseases.
The Impact of Mutations:
Mutations can have a range of effects, from neutral to detrimental to beneficial.
- Neutral mutations: Have no significant effect on the organism's phenotype.
- Deleterious mutations: Cause harm to the organism, potentially leading to disease or death. Many genetic diseases are caused by deleterious mutations.
- Beneficial mutations: Provide an advantage to the organism, increasing its fitness and chances of survival and reproduction. These mutations are the raw material for evolution.
Conclusion: The Ongoing Revolution in DNA Biology and Technology
DNA biology and technology are rapidly evolving fields. The advancements in our understanding of DNA replication, transcription, translation, and the mechanisms of mutation have paved the way for powerful technologies that are transforming medicine, agriculture, and many other aspects of our lives. From gene therapy to precision agriculture, the applications of DNA technology are virtually limitless. As our knowledge continues to expand, we can anticipate even more groundbreaking discoveries and applications in the future. Further research into DNA repair mechanisms, the complexities of gene regulation, and the development of more sophisticated gene-editing tools will continue to drive innovation and potentially address some of humanity's most pressing challenges. The ongoing exploration of the intricacies of DNA promises to unlock further secrets of life and offer solutions for a healthier and more sustainable future.
Latest Posts
Latest Posts
-
Solve The Triangle Round To The Nearest Tenth
Apr 24, 2025
-
Carl Jung Defined The Term Instinct As
Apr 24, 2025
-
Draw The Lewis Structure For A Dinitride 2 Ion
Apr 24, 2025
-
Concept Map Blood Groups And Transfusions
Apr 24, 2025
-
Is Force Decreased On A Incline Plane
Apr 24, 2025
Related Post
Thank you for visiting our website which covers about Dna Biology And Technology Transcription Translation And Mutation . We hope the information provided has been useful to you. Feel free to contact us if you have any questions or need further assistance. See you next time and don't miss to bookmark.