Chapter 16 Molecular Basis Of Inheritance
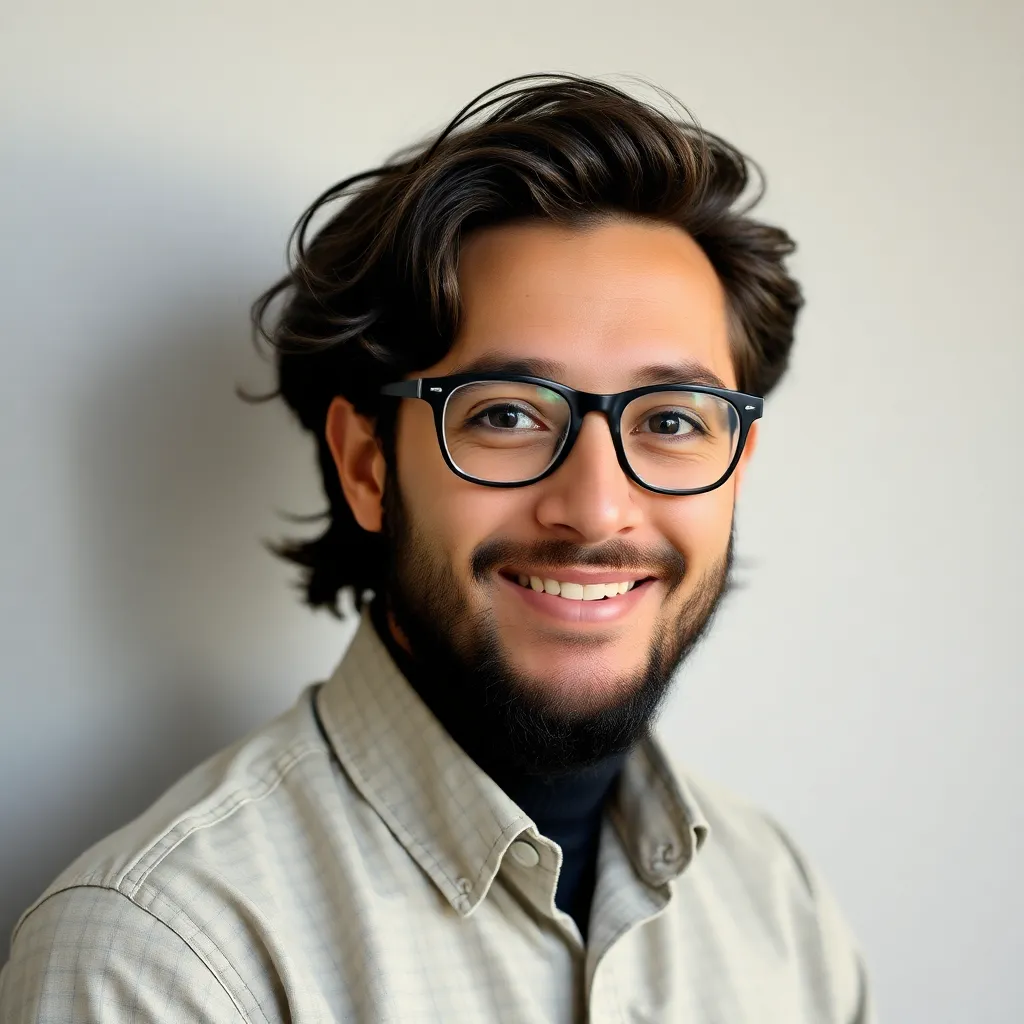
New Snow
Apr 25, 2025 · 6 min read

Table of Contents
Chapter 16: Molecular Basis of Inheritance – Unraveling the Secrets of Life
Understanding how traits are passed down through generations has been a central question in biology for centuries. The answer lies within the realm of molecular biology, specifically in the structure and function of DNA, the molecule that carries the genetic blueprint of life. This chapter delves into the molecular basis of inheritance, exploring the groundbreaking discoveries that revealed DNA's structure, its role in replication, and the mechanisms by which genetic information is expressed.
16.1: DNA as the Genetic Material
Before the structure of DNA was elucidated, scientists knew that genetic material was somehow responsible for heredity but remained uncertain about its exact nature. Several key experiments helped solidify DNA's role:
16.1.1 The Griffith Experiment: Transformation
Frederick Griffith's experiment in 1928 provided the first hint that genetic material could be transferred between bacteria. He worked with two strains of Streptococcus pneumoniae: a virulent, smooth strain (S) and a non-virulent, rough strain (R). He found that heat-killed S strain bacteria could transform live R strain bacteria into virulent S strain, suggesting that some transforming principle was responsible for this change.
16.1.2 Avery-MacLeod-McCarty Experiment: Identifying the Transforming Principle
Building upon Griffith's work, Oswald Avery, Colin MacLeod, and Maclyn McCarty identified the transforming principle as DNA. They systematically purified different components of the heat-killed S strain bacteria (proteins, RNA, DNA) and tested each for its ability to transform R strain bacteria. Only DNA was capable of transforming the R strain into the virulent S strain.
16.1.3 Hershey-Chase Experiment: Confirmation with Bacteriophages
Alfred Hershey and Martha Chase used bacteriophages (viruses that infect bacteria) to definitively confirm DNA as the genetic material. They labeled the phage's DNA with radioactive phosphorus (32P) and its protein coat with radioactive sulfur (35S). They found that the 32P label, but not the 35S label, entered the bacterial cells, further proving that DNA was the genetic material responsible for heredity.
16.2: The Structure of DNA: The Double Helix
The discovery of DNA's structure by James Watson and Francis Crick in 1953 was a monumental achievement in science. Their model, based on X-ray diffraction images by Rosalind Franklin and Maurice Wilkins, revealed DNA as a double helix:
16.2.1 Key Features of the DNA Double Helix
- Double-stranded: Two polynucleotide chains wound around each other.
- Antiparallel: The two strands run in opposite directions (5' to 3' and 3' to 5').
- Sugar-phosphate backbone: The outside of the helix is composed of alternating sugar (deoxyribose) and phosphate groups.
- Nitrogenous bases: The inside of the helix contains the nitrogenous bases: adenine (A), guanine (G), cytosine (C), and thymine (T).
- Base pairing: A pairs with T (two hydrogen bonds), and G pairs with C (three hydrogen bonds). This specific base pairing is crucial for DNA replication and transcription.
- Complementary strands: The sequence of bases on one strand dictates the sequence on the other strand due to base pairing rules.
16.3: DNA Replication: Making Copies of the Genome
The ability of DNA to replicate itself accurately is essential for inheritance. DNA replication is a semi-conservative process, meaning that each new DNA molecule consists of one original strand and one newly synthesized strand.
16.3.1 The Replication Process
- Initiation: Replication begins at specific sites on the DNA molecule called origins of replication.
- Unwinding: The enzyme helicase unwinds the DNA double helix, creating a replication fork.
- Primer synthesis: Short RNA primers are synthesized by primase, providing a starting point for DNA polymerase.
- Elongation: DNA polymerase adds nucleotides to the 3' end of the RNA primer, synthesizing new DNA strands that are complementary to the template strands. Leading strand synthesis is continuous, while lagging strand synthesis is discontinuous, resulting in Okazaki fragments.
- Joining: DNA ligase joins the Okazaki fragments together to form a continuous lagging strand.
- Termination: Replication terminates when the entire DNA molecule has been duplicated.
16.3.2 Proofreading and Repair
DNA polymerase has a proofreading function that minimizes errors during replication. Various repair mechanisms also exist to correct any remaining errors or damage to the DNA molecule.
16.4: From DNA to Protein: The Central Dogma
The central dogma of molecular biology describes the flow of genetic information from DNA to RNA to protein:
16.4.1 Transcription: DNA to RNA
Transcription is the process of synthesizing RNA from a DNA template. RNA polymerase binds to a promoter region on the DNA, unwinds the DNA, and synthesizes a complementary RNA molecule. The RNA molecule is processed before it leaves the nucleus (in eukaryotes). This processing includes capping, splicing (removal of introns and joining of exons), and polyadenylation.
16.4.2 Translation: RNA to Protein
Translation is the process of synthesizing a protein from an mRNA template. This occurs on ribosomes. Transfer RNA (tRNA) molecules carry amino acids to the ribosome, where they are added to the growing polypeptide chain according to the mRNA sequence. The genetic code dictates which codon (three-nucleotide sequence) corresponds to which amino acid.
16.4.3 The Genetic Code
The genetic code is a triplet code, meaning that each codon consists of three nucleotides. It is also redundant (more than one codon can code for the same amino acid) and nearly universal (the same code is used by almost all organisms). The start codon (AUG) initiates translation, while stop codons (UAA, UAG, UGA) terminate translation.
16.5: Mutations: Changes in the Genetic Material
Mutations are changes in the DNA sequence. They can be spontaneous or induced by mutagens (e.g., radiation, certain chemicals). Mutations can have various effects, ranging from no effect to lethal consequences.
16.5.1 Types of Mutations
- Point mutations: Changes in a single nucleotide. These can be substitutions (one base is replaced with another), insertions (a base is added), or deletions (a base is removed).
- Frameshift mutations: Insertions or deletions that shift the reading frame of the gene, often resulting in a non-functional protein.
- Chromosomal mutations: Larger-scale changes involving entire chromosomes or segments of chromosomes. These can include deletions, duplications, inversions, and translocations.
16.6: Regulation of Gene Expression
Gene expression is the process by which genetic information is used to synthesize a functional gene product. The expression of genes is tightly regulated to ensure that proteins are produced only when and where they are needed.
16.6.1 Mechanisms of Gene Regulation
Gene regulation can occur at many levels, including:
- Transcriptional control: Regulation of the initiation of transcription. This involves transcription factors that bind to DNA and either enhance or repress transcription.
- Post-transcriptional control: Regulation of mRNA processing, stability, and translation.
- Post-translational control: Regulation of protein activity after translation. This can involve protein modification, degradation, or interaction with other molecules.
16.7: Further Exploration: Epigenetics
Epigenetics is the study of heritable changes in gene expression that do not involve changes to the underlying DNA sequence. These changes can be caused by various factors, including DNA methylation and histone modification. Epigenetic changes can affect gene expression over long periods, even across generations. Understanding epigenetics provides a crucial layer of complexity to our understanding of inheritance and gene regulation.
This chapter provides a comprehensive overview of the molecular basis of inheritance. The intricate details of DNA structure, replication, transcription, and translation, along with the concepts of mutations and gene regulation, paint a rich picture of the sophisticated mechanisms underlying life itself. The study of molecular biology continues to reveal new and exciting insights into the complexities of inheritance, pushing the boundaries of our understanding of life's fundamental processes. Further research continues to explore the interactions between genes, environment, and epigenetic influences, shaping our understanding of how traits are inherited and expressed.
Latest Posts
Latest Posts
-
Assume That When Human Resource Managers Are Randomly Selected
Apr 25, 2025
-
Which Of The Following Statements Regarding Striated Muscle Is Correct
Apr 25, 2025
-
Unit 7 Natural Selection Ap Biology Exam Review
Apr 25, 2025
-
40 Healing Scriptures By Dodie Osteen
Apr 25, 2025
-
Match The Definitions To The Appropriate Terms
Apr 25, 2025
Related Post
Thank you for visiting our website which covers about Chapter 16 Molecular Basis Of Inheritance . We hope the information provided has been useful to you. Feel free to contact us if you have any questions or need further assistance. See you next time and don't miss to bookmark.