Ch 18 Regulation Of Gene Expression
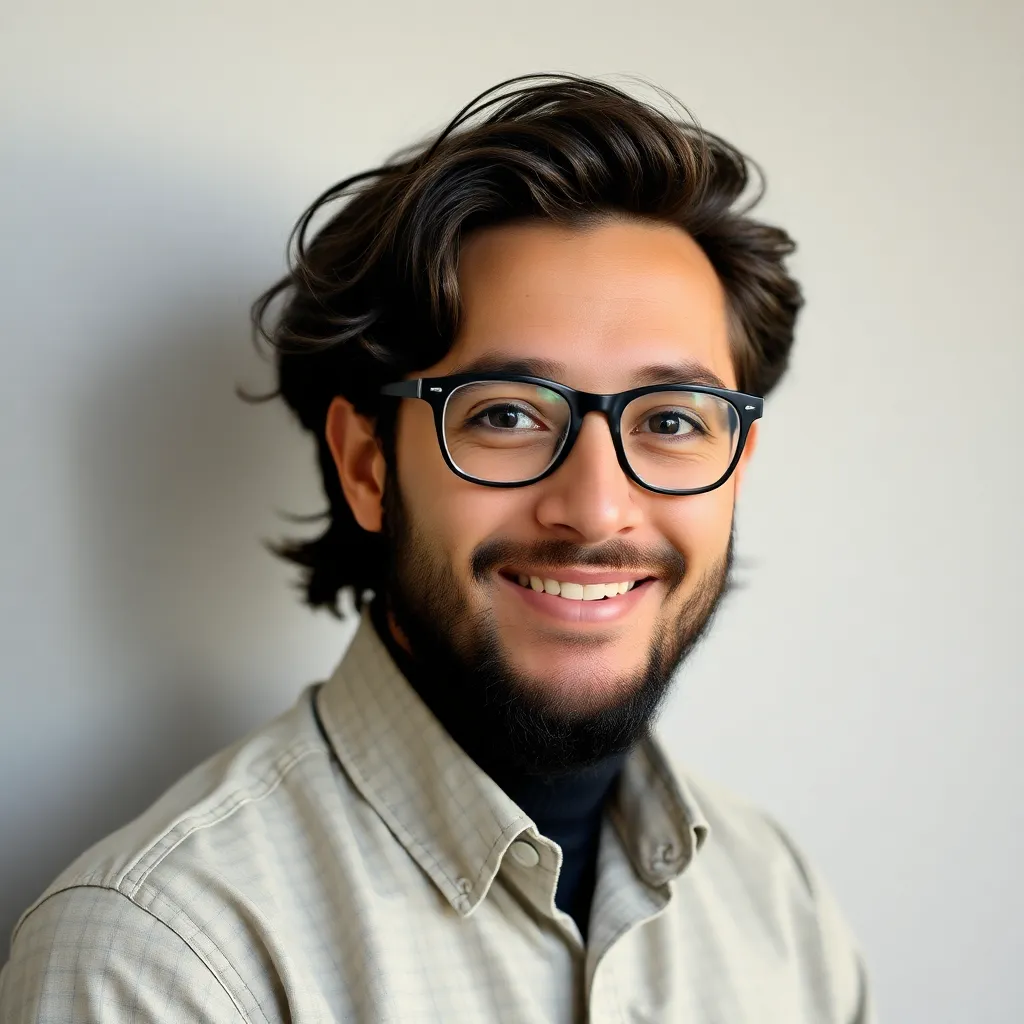
New Snow
Apr 24, 2025 · 6 min read

Table of Contents
Chapter 18: Regulation of Gene Expression: A Deep Dive
Gene expression, the intricate process by which information encoded within a gene is translated into a functional product, such as a protein, is a tightly controlled affair. Understanding the regulation of gene expression is crucial to comprehending the complexities of cellular function, development, and disease. This chapter delves deep into the mechanisms that govern this crucial process, exploring the multifaceted layers of control operating in both prokaryotic and eukaryotic cells.
Prokaryotic Gene Regulation: A Simple Yet Elegant System
Prokaryotic organisms, such as bacteria, have evolved efficient mechanisms for regulating gene expression, primarily focused on maximizing resource utilization and responding rapidly to environmental changes. The classic example, the lac operon in E. coli, beautifully illustrates this principle.
The lac Operon: A Paradigm of Operon Regulation
The lac operon controls the expression of genes involved in lactose metabolism. When lactose is absent, the lac repressor protein, encoded by the lacI gene, binds to the operator region, preventing RNA polymerase from transcribing the structural genes (lacZ, lacY, lacA). This represents negative regulation, where a protein actively represses transcription.
Key components of the lac operon:
- Promoter: The binding site for RNA polymerase.
- Operator: The binding site for the lac repressor protein.
- Structural genes: Genes encoding proteins involved in lactose metabolism (lacZ, β-galactosidase; lacY, lactose permease; lacA, transacetylase).
- lacI gene: Encodes the lac repressor protein.
However, when lactose is present, it acts as an inducer, binding to the repressor protein and causing a conformational change that prevents it from binding to the operator. This allows RNA polymerase to transcribe the structural genes, leading to lactose metabolism.
Catabolite Repression: Preference for Glucose
The lac operon also demonstrates catabolite repression, a regulatory mechanism that prioritizes glucose as a carbon source. In the presence of both glucose and lactose, glucose metabolism is favored, resulting in low levels of lac operon expression even when lactose is present. This is mediated by cAMP levels; low glucose levels lead to high cAMP, which activates CAP (catabolite activator protein), enhancing RNA polymerase binding to the promoter.
Other Prokaryotic Regulatory Mechanisms
Beyond operons, prokaryotes utilize other strategies for gene regulation including:
- Attenuation: Transcriptional termination controlled by the formation of secondary structures in the mRNA. This mechanism is frequently found in genes involved in amino acid biosynthesis.
- Riboswitches: RNA structures within the mRNA that bind to small molecules, influencing transcription or translation.
- Two-component regulatory systems: These systems involve a sensor kinase that detects environmental signals and a response regulator that modulates gene expression.
Eukaryotic Gene Regulation: A Multi-Layered Approach
Eukaryotic gene regulation is considerably more complex than in prokaryotes, involving multiple layers of control acting at various stages, from chromatin remodeling to post-translational modification of proteins.
Chromatin Remodeling: Accessing the DNA
The DNA in eukaryotic cells is packaged into chromatin, a complex of DNA and proteins (histones). Chromatin structure plays a crucial role in regulating gene accessibility to the transcriptional machinery.
- Histone modification: Chemical modifications of histone tails (e.g., acetylation, methylation, phosphorylation) can alter chromatin structure, either promoting or repressing transcription. Histone acetyltransferases (HATs) add acetyl groups, generally relaxing chromatin structure and increasing transcription, while histone deacetylases (HDACs) remove acetyl groups, compacting chromatin and repressing transcription.
- DNA methylation: The addition of methyl groups to DNA bases, typically cytosine, often leads to gene silencing. This is a crucial mechanism in development and genomic imprinting.
- Chromatin remodeling complexes: These large protein complexes use ATP to reposition nucleosomes, altering chromatin structure and affecting gene expression.
Transcriptional Regulation: Orchestrating the Transcriptional Machinery
Transcriptional regulation in eukaryotes involves a vast array of proteins, including:
- Transcription factors: These proteins bind to specific DNA sequences (cis-regulatory elements) in the promoter and enhancer regions, influencing the recruitment and activity of RNA polymerase. Activators enhance transcription, while repressors inhibit it.
- RNA polymerase II: The main enzyme responsible for transcribing protein-coding genes. Its activity is regulated by numerous factors, including general transcription factors and specific transcription factors.
- Enhancers and silencers: These are DNA sequences located far from the promoter that can either enhance or repress transcription. They function by interacting with transcription factors and the transcriptional machinery through DNA looping.
Post-Transcriptional Regulation: Fine-Tuning Gene Expression
Gene expression is further regulated after transcription through several mechanisms:
- RNA processing: This includes splicing, capping, and polyadenylation of pre-mRNA molecules. Alternative splicing allows for the production of multiple protein isoforms from a single gene.
- RNA stability: The lifespan of mRNA molecules is crucial in determining protein levels. mRNA degradation is influenced by various factors, including the presence of specific sequences in the 3' untranslated region (3'UTR).
- RNA interference (RNAi): Small RNA molecules (microRNAs and siRNAs) can bind to complementary sequences in mRNA, leading to either mRNA degradation or translational repression. This is a powerful mechanism for regulating gene expression, particularly in development and defense against viruses.
- Translational regulation: The translation of mRNA into protein can be regulated by various factors, including the availability of initiation factors, the presence of inhibitory proteins, and the structure of the 5' and 3' untranslated regions (UTRs) of the mRNA.
Post-Translational Regulation: The Final Control
Even after protein synthesis, gene expression can be regulated through:
- Protein modification: Post-translational modifications, such as phosphorylation, glycosylation, and ubiquitination, can alter protein activity, localization, and stability.
- Protein degradation: Proteins can be targeted for degradation by the ubiquitin-proteasome system, controlling their abundance and activity.
Integrating Signals: A Coordinated Response
Eukaryotic cells must integrate a multitude of signals to respond appropriately to their environment. This integration occurs at multiple levels of gene regulation, allowing for a coordinated and adaptable response. For instance, signals from growth factors can activate specific transcription factors, leading to the expression of genes involved in cell growth and division. Simultaneously, other signals might influence chromatin remodeling or RNA processing, fine-tuning the overall cellular response.
Dysregulation and Disease: When Control Breaks Down
Errors in gene regulation are implicated in a wide range of diseases, including cancer, developmental disorders, and neurological conditions. For example, mutations in transcription factors or chromatin remodeling proteins can disrupt gene expression patterns, contributing to uncontrolled cell growth in cancer. Similarly, defects in RNA processing or RNAi pathways can lead to various developmental abnormalities. Understanding the mechanisms of gene regulation is therefore essential for developing therapies for these diseases.
Conclusion: A Dynamic and Complex Process
The regulation of gene expression is a multifaceted and dynamic process crucial for all life forms. Prokaryotes rely on efficient, rapid mechanisms like operons, while eukaryotes utilize a more complex, multi-layered system involving chromatin remodeling, transcriptional regulation, post-transcriptional regulation, and post-translational modification. Dysregulation of these processes can have profound consequences, highlighting the critical role of gene expression control in health and disease. Further research continues to uncover the intricate details of this fundamental biological process, providing valuable insights into the mechanisms driving cellular function and disease pathogenesis. The study of gene regulation remains a vibrant and essential area of biological research, promising breakthroughs in understanding life's complexities and developing new therapeutic strategies.
Latest Posts
Related Post
Thank you for visiting our website which covers about Ch 18 Regulation Of Gene Expression . We hope the information provided has been useful to you. Feel free to contact us if you have any questions or need further assistance. See you next time and don't miss to bookmark.