Ap Bio Chapter 16 Reading Guide
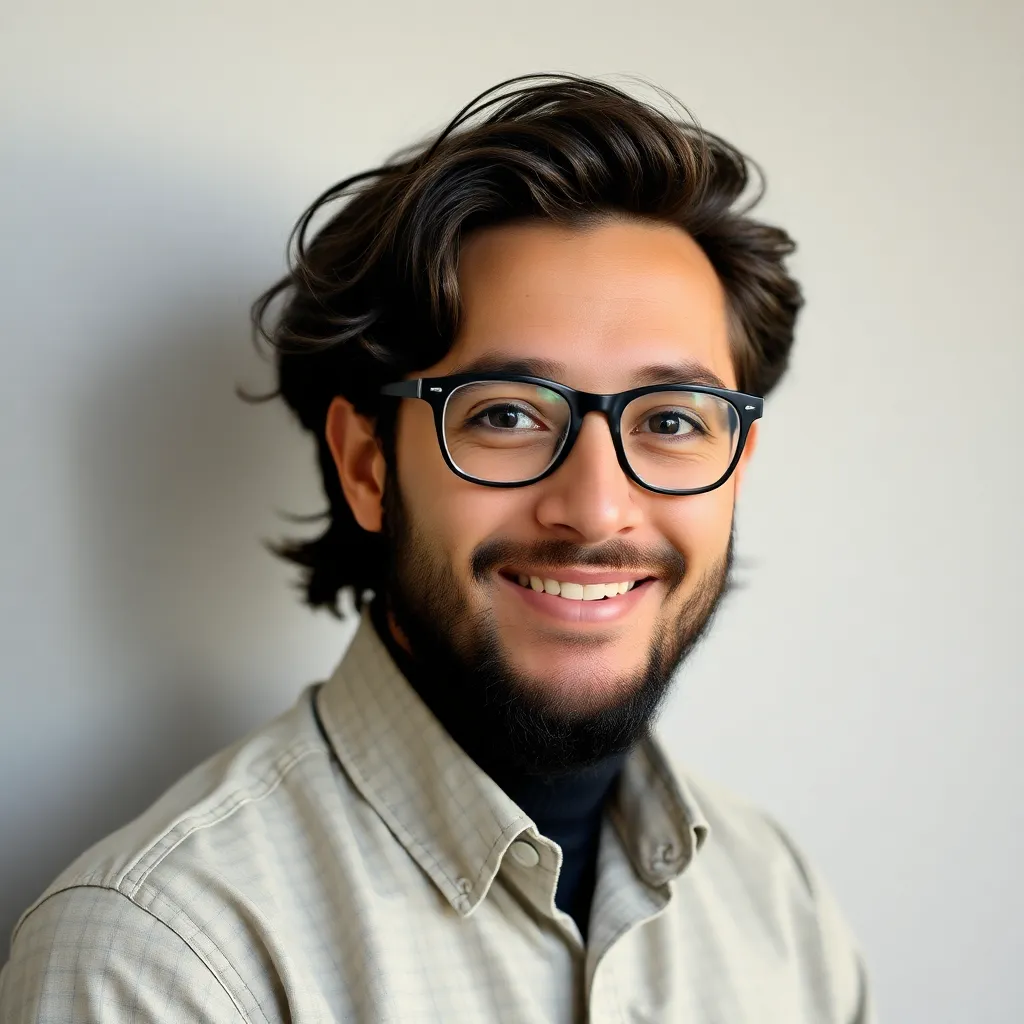
New Snow
Apr 21, 2025 · 6 min read

Table of Contents
AP Bio Chapter 16 Reading Guide: A Deep Dive into Molecular Basis of Inheritance
Chapter 16 of your AP Biology textbook delves into the fascinating world of molecular genetics, focusing on the structure and function of DNA – the molecule of heredity. Understanding this chapter is crucial for success in the AP Biology exam. This comprehensive guide will break down the key concepts, providing you with a robust framework for mastering this vital chapter.
I. DNA: The Blueprint of Life
The central dogma of molecular biology – DNA → RNA → protein – underpins this entire chapter. Let's start by solidifying our understanding of DNA's structure and function.
A. The Structure of DNA: A Double Helix
Watson and Crick's groundbreaking discovery revealed DNA's double helix structure, a crucial breakthrough in understanding inheritance. This structure, with its antiparallel strands and complementary base pairing (adenine with thymine, guanine with cytosine), allows for DNA replication and the transmission of genetic information across generations.
- Key terms to master: deoxyribose sugar, phosphate backbone, nitrogenous bases, antiparallel, complementary base pairing, hydrogen bonds.
- Understanding the significance: The specific base pairing ensures accurate replication. The double helix structure itself provides stability and protection for the genetic code.
B. Chargaff's Rules and Their Implications
Erwin Chargaff's observations, showing that the amounts of adenine and thymine are equal, as are the amounts of guanine and cytosine in DNA, provided critical evidence supporting Watson and Crick's model. These rules highlight the importance of complementary base pairing.
- Key terms to master: Chargaff's rules, purines, pyrimidines.
- Understanding the significance: These rules are fundamental to understanding DNA replication and the mechanism of heredity.
II. DNA Replication: Faithful Copying of the Genetic Material
The ability of DNA to replicate itself accurately is essential for the transmission of genetic information. This section focuses on the process of DNA replication, highlighting its semi-conservative nature.
A. The Meselson-Stahl Experiment: Evidence for Semi-Conservative Replication
The Meselson-Stahl experiment elegantly demonstrated that DNA replication is semi-conservative – each new DNA molecule consists of one original strand and one newly synthesized strand. This experiment used isotopes of nitrogen to track DNA replication.
- Key terms to master: semi-conservative replication, conservative replication, dispersive replication, density gradient centrifugation, isotopes.
- Understanding the significance: This experiment definitively proved the mechanism of DNA replication.
B. The Enzymes and Proteins Involved in DNA Replication
DNA replication is a complex process involving a multitude of enzymes and proteins working in coordination. This includes:
-
Helicases: Unwind the DNA double helix.
-
Single-strand binding proteins (SSBs): Prevent the separated strands from re-annealing.
-
Topoisomerases (e.g., DNA gyrase): Relieve the torsional strain ahead of the replication fork.
-
Primase: Synthesizes RNA primers to initiate DNA synthesis.
-
DNA polymerase III: The primary enzyme responsible for DNA synthesis, adding nucleotides to the 3' end of the growing strand.
-
DNA polymerase I: Removes RNA primers and replaces them with DNA.
-
Ligase: Joins Okazaki fragments on the lagging strand.
-
Key terms to master: replication fork, leading strand, lagging strand, Okazaki fragments, RNA primer, 3' to 5' exonuclease activity (proofreading).
-
Understanding the significance: Each enzyme plays a specific and crucial role in ensuring accurate and efficient DNA replication. Understanding their functions and interactions is key.
C. The Leading and Lagging Strands: Differences in Synthesis
DNA polymerase can only add nucleotides to the 3' end of a growing strand. This leads to the formation of a leading strand (synthesized continuously) and a lagging strand (synthesized discontinuously in Okazaki fragments).
- Key terms to master: leading strand, lagging strand, Okazaki fragments.
- Understanding the significance: The discontinuous synthesis of the lagging strand adds complexity to the replication process but ensures complete replication of both strands.
III. DNA Repair Mechanisms: Maintaining the Integrity of the Genome
Errors in DNA replication are inevitable, but cells have evolved sophisticated mechanisms to repair these errors and maintain the integrity of the genome.
A. Types of DNA Damage
Various factors can cause DNA damage, including:
-
Spontaneous mutations: Errors during replication.
-
UV radiation: Formation of thymine dimers.
-
Chemical mutagens: Modification of DNA bases.
-
Ionizing radiation: Double-strand breaks.
-
Key terms to master: thymine dimers, mismatch repair, excision repair.
-
Understanding the significance: DNA damage can have severe consequences, including cell death or cancer. Repair mechanisms are crucial for maintaining genomic stability.
B. Repair Mechanisms
Cells employ various DNA repair mechanisms, including:
-
Mismatch repair: Corrects errors made during replication.
-
Excision repair (base excision repair and nucleotide excision repair): Removes damaged or modified bases.
-
Double-strand break repair: Repairs breaks in both DNA strands (often through homologous recombination or non-homologous end joining).
-
Key terms to master: mismatch repair, excision repair, base excision repair, nucleotide excision repair, homologous recombination, non-homologous end joining.
-
Understanding the significance: These mechanisms are essential for preventing mutations and maintaining the integrity of the genome.
IV. Telomeres and Telomerase: Protecting Chromosome Ends
Telomeres are repetitive DNA sequences at the ends of chromosomes that protect them from degradation and fusion. Telomerase is an enzyme that maintains telomere length.
A. Telomere Shortening and Aging
With each round of replication, telomeres shorten slightly. This shortening is linked to cellular aging and senescence.
- Key terms to master: telomeres, telomerase, senescence.
- Understanding the significance: Telomere shortening plays a role in aging and age-related diseases.
B. Telomerase Activity in Cancer Cells
Telomerase is often reactivated in cancer cells, allowing them to maintain telomere length and achieve immortality.
- Key terms to master: telomerase activity, cancer cells, immortality.
- Understanding the significance: Telomerase activity is a hallmark of cancer cells and a potential target for cancer therapies.
V. PCR: Amplifying DNA in the Lab
Polymerase chain reaction (PCR) is a powerful technique used to amplify specific DNA sequences in vitro.
A. The PCR Process: Cycling Through Temperature Stages
PCR involves repeated cycles of heating and cooling, allowing for the exponential amplification of a target DNA sequence.
- Key terms to master: polymerase chain reaction (PCR), primers, Taq polymerase, thermal cycler.
- Understanding the significance: PCR is a fundamental technique used in many areas of biology and medicine.
B. Applications of PCR
PCR has numerous applications, including:
-
Forensic science: DNA fingerprinting.
-
Medical diagnostics: Detecting pathogens.
-
Genetic research: Cloning genes.
-
Gene therapy: Introducing genes into cells.
-
Key terms to master: DNA fingerprinting, medical diagnostics, cloning.
-
Understanding the significance: The versatility of PCR makes it an invaluable tool in numerous fields.
VI. Gel Electrophoresis: Separating DNA Fragments
Gel electrophoresis is a technique used to separate DNA fragments based on their size.
A. The Process of Gel Electrophoresis
DNA fragments are loaded into a gel and subjected to an electric field. Smaller fragments migrate faster than larger fragments.
- Key terms to master: gel electrophoresis, agarose gel, DNA ladder, electrophoresis chamber.
- Understanding the significance: Gel electrophoresis is crucial for visualizing and analyzing DNA fragments.
B. Applications of Gel Electrophoresis
Gel electrophoresis is used in conjunction with many techniques, including PCR, to analyze DNA fragments. Applications include:
-
DNA fingerprinting: Analyzing DNA fragments to identify individuals.
-
Restriction fragment length polymorphism (RFLP) analysis: Analyzing DNA fragments produced by restriction enzymes.
-
Key terms to master: RFLP analysis, restriction enzymes.
-
Understanding the significance: Gel electrophoresis is an indispensable technique in many molecular biology applications.
This detailed guide provides a comprehensive overview of the key concepts covered in AP Biology Chapter 16. Remember to actively engage with the material, utilize practice problems, and seek clarification from your teacher or peers when needed. Mastering this chapter will significantly enhance your understanding of molecular genetics and improve your performance on the AP Biology exam. Good luck!
Latest Posts
Latest Posts
-
How To Sale Anything To Anybody Pdf
Apr 21, 2025
-
What Are Striations On A Bullet
Apr 21, 2025
-
Unit 11 Volume And Surface Area Homework 5
Apr 21, 2025
-
The Royal Fruit Company Produces Two
Apr 21, 2025
-
Contrato De Prestamo De Dinero Word
Apr 21, 2025
Related Post
Thank you for visiting our website which covers about Ap Bio Chapter 16 Reading Guide . We hope the information provided has been useful to you. Feel free to contact us if you have any questions or need further assistance. See you next time and don't miss to bookmark.